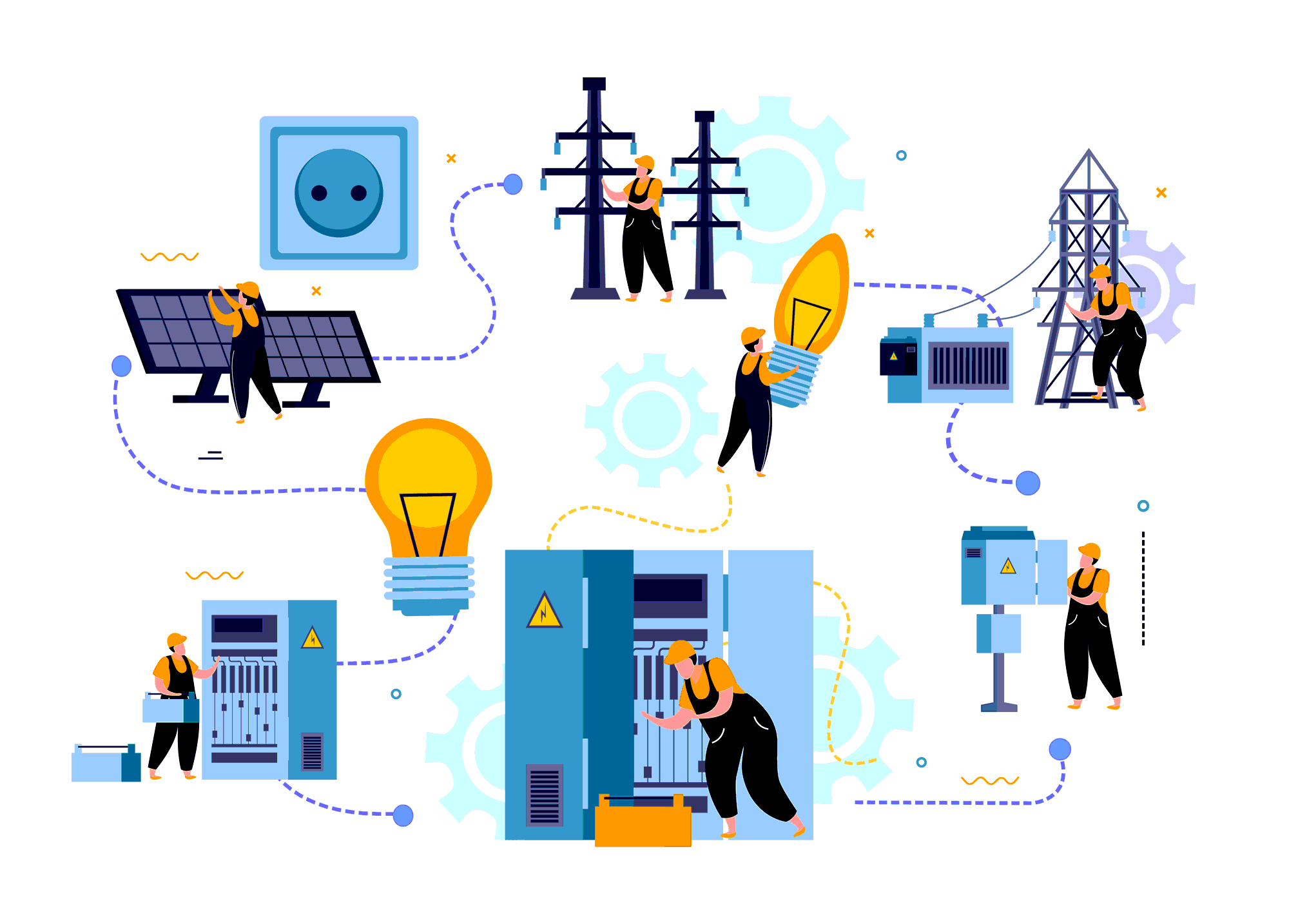
Energy Storage in Electrical Engineering: Innovations and Applications for a Sustainable Future
The demand for energy is constantly increasing, and as a result, the power grid is facing new challenges in ensuring a stable supply of electricity. Energy storage systems have emerged as a solution to address these challenges, enabling the integration of renewable energy sources, improving grid stability, and reducing energy costs. This article will explore the latest innovations in energy storage technology, their applications in the power grid and renewable energy systems, and the future of energy storage research and development.
Overview of Energy Storage Systems
Energy storage systems (ESS) are designed to store electrical energy in various forms, including mechanical, electrochemical, and thermal energy. ESS play a critical role in ensuring grid stability, balancing the supply and demand of electricity, and reducing energy costs. ESS can be classified into three categories based on their power and energy capacities:
- Short-term energy storage systems: These systems are designed to provide power for a few seconds to a few hours, such as supercapacitors, flywheels, and batteries.
- Medium-term energy storage systems: These systems are designed to provide power for a few hours to a few days, such as pumped hydro storage and compressed air energy storage.
- Long-term energy storage systems: These systems are designed to provide power for several days to weeks or longer, such as hydrogen storage and thermal energy storage.
Advancements in Energy Storage Technologies
The energy storage industry has witnessed significant advancements in technology in recent years, with a focus on improving the performance, reliability, and cost-effectiveness of ESS. Lithium-ion batteries have dominated the energy storage market due to their high energy density, long cycle life, and low self-discharge rate. However, other battery technologies, such as flow batteries, sodium-ion batteries, and solid-state batteries, are also gaining popularity due to their potential to overcome some of the limitations of lithium-ion batteries, such as safety concerns and limited availability of raw materials.
In addition to battery technologies, other ESS, such as hydrogen storage, thermal energy storage, and pumped hydro storage, are also being developed and deployed in various applications. For example, hydrogen storage is being explored as a potential energy storage solution for the transportation sector, while thermal energy storage is being used for heating and cooling applications in buildings.
Applications of Energy Storage Systems
Energy storage systems have a wide range of applications in the power grid and renewable energy systems, including:
- Integration of renewable energy sources: ESS enable the integration of variable renewable energy sources, such as wind and solar, into the power grid by smoothing out the fluctuations in power output and providing a stable supply of electricity.
- Peak shaving: ESS can be used to reduce peak demand on the power grid, which can help avoid the need for building new power plants and transmission lines.
- Microgrids: ESS can be used to create microgrids, which are self-sufficient power systems that can operate independently of the main power grid. Microgrids can increase grid resiliency and reduce the risk of power outages.
- Backup power: ESS can be used as backup power for critical facilities, such as hospitals, data centers, and military bases.
- Frequency regulation: ESS can provide frequency regulation services to the power grid, which help maintain a stable frequency and prevent blackouts.
Performance and Cost-effectiveness of Different Energy Storage Devices
The performance and cost-effectiveness of ESS are critical factors that determine their suitability for different applications. The performance of an ESS is measured by its power and energy capacities, efficiency, round-trip efficiency, cycle life, and response time. The cost-effectiveness of an ESS is determined by its capital cost, operating cost, and lifetime cost, which includes the initial capital cost, replacement cost, and maintenance cost.
In general, the choice of an ESS technology depends on the specific application, the required power and energy capacities, and the budget. Some ESS technologies are better suited for high-power, short-duration applications, while others are better suited for low-power, long-duration applications. For example, lithium-ion batteries are well-suited for short-duration applications, such as providing backup power for data centers or smoothing out power fluctuations in a wind farm. On the other hand, flow batteries are better suited for long-duration applications, such as storing solar energy for use during the night.
Other important factors to consider when choosing an ESS technology include safety, environmental impact, and scalability. Safety is a critical consideration, especially for large-scale ESS installations. Lithium-ion batteries, for example, have a higher risk of thermal runaway and fire than other ESS technologies, such as flow batteries or pumped hydro storage. Environmental impact is another important consideration, as some ESS technologies require rare or toxic materials, such as lithium or lead-acid batteries. Finally, scalability is important for ESS technologies, as they may need to be scaled up or down to meet changing power demands.
Conclusion
In summary, ESS technologies play a critical role in the development of a sustainable and resilient energy system. The choice of an ESS technology depends on the specific application, the required power and energy capacities, and the budget. It is important to consider factors such as performance, cost-effectiveness, safety, environmental impact, and scalability when selecting an ESS technology.
Engineering Topics Electrical Engineering